Biomass gasification is a thermochemical process that converts solid biomass fuel into producer gas fuel in a gasifier reactor. Unlike combustion, gasification requires much less air than stoichiometric air for combustion. The effective equivalence ratio for biomass gasification lies between 0.20 and 0.40 [1]. It is a ratio between the actual air-fuel ratio for gasification and the stoichiometric air-fuel ratio for combustion. When air is used as a gasification agent, the producer gas contains CO, CO2, H2, CH4, and N2. The presence of CO, H2, and CH4 leads to combustible producer gas. Cleaner combustion of producer gas to replace direct combustion of solid biomass [2] has driven increasing attention on biomass gasification in recent years
In a downdraft gasifier, the biomass feedstock and air enter the gasifier from the top of the reactor. Biomass processing includes drying, pyrolysis, oxidation (combustion), and reduction (gasification). Producer gas exits the gasifier at the lower part of the reactor, as shown by a schematic diagram of the downdraft gasifier in Figure 1a. A typical axial temperature profile of a downdraft gasifier for wood gasification [3] is shown in Figure 1b. During reduction, combustible gases CO, H2, and CH4 are formed through exothermic reactions of Boudouard, eq. (1) and Water-Gas, eq. (2), as well as endothermic reactions of Water-Gas Shift, eq. (3) and Methane Formation, eq. (4) [3].
(1)
(2)
(3)
(4)
Downdraft gasifier: scheme of gasification (a) and axial temperature profile (b)
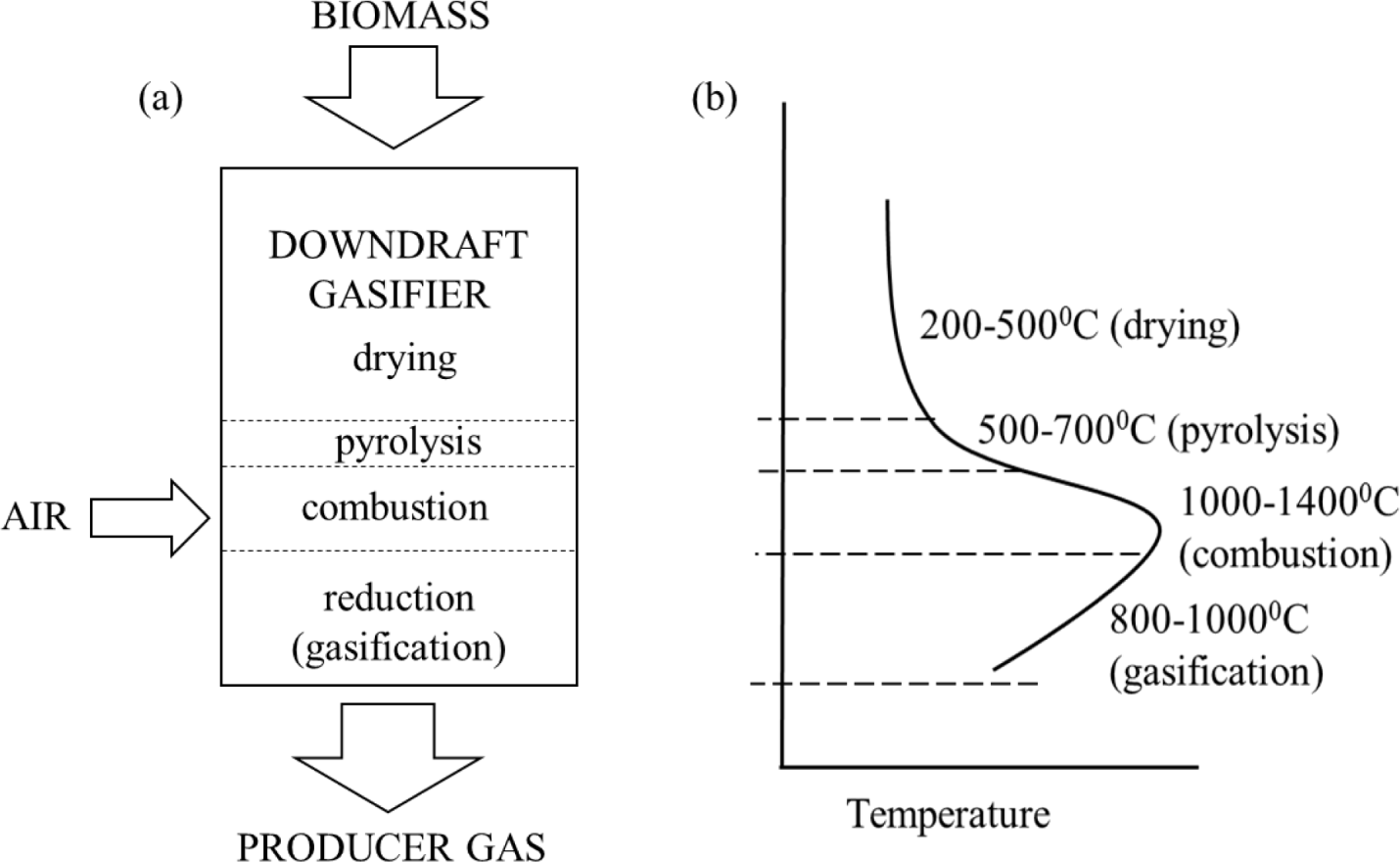
The performance of the gasifier can be affected by many factors, such as equivalence ratio (ø) and gasification temperature. Increasing the equivalence ratio improves the oxidation process and releases more heat, increasing gasification temperature [4]. The tar cracking process occurs better at higher gasification temperatures [5] which increases producer gas yields and higher heating value of the producer gas (HHVg). Increasing HHVg with increasing gasification temperature is a result of improving the volume fraction of H2 and CO in the producer gas [6].
Research on biomass gasification has been reported by many researchers worldwide. Different biomasses, especially agricultural and forestry waste, have been explored as gasification feedstock. Balu & Chung [7] and Perez et al. [8] used pine wood as the feedstock in a downdraft gasifier. The operating conditions and design parameters affected gasifier performance significantly. Sheth & Babu [9] utilised waste of sesame wood from the furniture industry and found that the optimum equivalence ratio for gasification of sesame wood was 0.205. Yin et al. [10] performed gasification of peach prunings. They stated that prunings with a particle size of 1–2 cm were favourable for gasification in the 25 kg/h fixed-bed downdraft gasifier used in their work. Other researchers have also conducted works on the gasification of wood feedstock. The lower heating value of the producer gas from the gasification of the wood chips was 1100−1200 kcal/Nm3 (4.60−5.02 MJ/Nm3) with a tar content of 3.9–4.4 g/Nm3 for an air ratio of 0.3−0.35 [11]. Tar content in producer gas can be reduced by the method of two-stage air supply into the gasifier. The tar content of producer gas from eucalyptus wood gasification was reduced by 87% using this method [12].
On the other hand, downdraft gasifiers can also use non-woody biomasses as feedstock. These include oil palm frond, corn straw, groundnut and cashew nut shell, hazelnut shell, rice husk, and sawdust. Bhoi and Singh [13] found that the gasification of groundnut shell has lower efficiency than the gasification of babul wood. Gasifier selection and design criteria for oil palm frond were studied by Guangul et al. [14]. Gasification of oil palm frond using preheated air made it possible to increase the performance of a single throat downdraft gasifier [15]. Corn straw gasification in a downdraft gasifier was reported by Gai and Dong [16]. They revealed that over the ranges of the equivalence ratio examined, higher or lower equivalence ratios both degraded the quality of gas and gasification efficiency of corn straw. The potential of cashew nut shell [17] and hazelnut shell [18] as gasifier feedstock were also investigated. Based on the investigation results, both shells have a good potential as a gasifier feedstock. Rice husk and sawdust are other non-woody biomasses that received increasing attention as gasifier feedstock. Susastriawan et al. [19] investigated the effect of tuyere grate distance above the grate on the performance of a downdraft gasifier fed by rice husk. The results indicated that the tuyere distance above the grate impacts the gasifier performance. A lower heating value of 4.44 MJ/Nm3 and tar content of 1.34 g/Nm3 of rice husk producer gas at an equivalence ratio of 0.211 was reported by Ma et al.[20]. Yoon et al. [21] reported producer gas from rice husk gasification has been used successfully as a fuel for a 10 kW reciprocating engine. Meanwhile, Wander et al. [22] developed an open-top downdraft gasifier with a processing capacity of around 12 kg/h. The gasifier was operated successfully using sawdust as a feedstock.
In the present work, sawdust waste is gasified in a small-scale downdraft gasifier at an equivalence ratio of 0.15, 0.20, and 0.25, and air tuyere position at 260, 330, and 300 mm above the grate. No research on the effect of air tuyere position above a grate and equivalence ratio on the thermal performance of the small-scale downdraft gasifier fed by sawdust has been reported so far. The present work aims to investigate the axial temperature profile of the reactor, the volume percentage of CO, H2, and CH4, the higher heating value of the producer gas (HHVg), and the cold gas efficiency (CGE) of the gasifier.
The present work investigates teak sawdust waste collected from the wood furniture industry at Bantul Regency in Yogyakarta Province. Before being used as feedstock for the experimental downdraft gasifier, the sawdust is analysed for its proximate and ultimate properties.
Proximate and ultimate analyses are performed at Tekmira, Bandung. Table 1 shows a photo of the sawdust and the results of the analyses. The results are presented in weight percentage (% wt) on air dried basis (adb). The sawdust is most likely to contain nitrogen. However, the nitrogen content is undedicated during the ultimate test, possibly due to the sample's very small nitrogen content.
Proximate and ultimate analysis of the sawdust
Teak sawdust |
Proximate |
[% wt] adb |
Ultimate |
[% wt] adb |
---|---|---|---|---|
Fixed Carbon |
13.29 |
C |
44.99 |
|
Volatile matter |
71.48 |
H |
6.68 |
|
Ash |
1.97 |
O |
45.62 |
|
Moisture |
13.26 |
N |
- |
|
S |
0.74 |
|||
HHVf [MJ/kg] |
17.577 |
Figure 2 displays a photo and a schematic diagram of the experimental setup used in the present work. The reactor, made of steel, has an internal diameter of 300 mm and a height of 725 mm (from the grate to the top). The reactor is insulated with glass wool with a thickness of 50 mm. The sawdust feedstock is fed into the gasifier at the top of the reactor. Gasification air is supplied using a blower through three series of tuyeres located at 260, 330, and 400 mm above the grate. Each tuyere series has three tuyeres 1 inch in diameter. The air flow rate is controlled using a valve and is measured using a rotameter. Meanwhile, six K-type thermocouples are inserted into the reactor at 50, 120, 190, 260, 330, and 400 mm above the grate. Temperature measurement data are logged using data logger OMRON ZR-RX45. The producer gas is sucked from the lower part of the reactor using a suction fan and transferred to the flare. Gasification is initiated by torching the reactor feedstock through an ignition port located 120 mm above the grate.
Photo and schematic diagram of the experimental setup
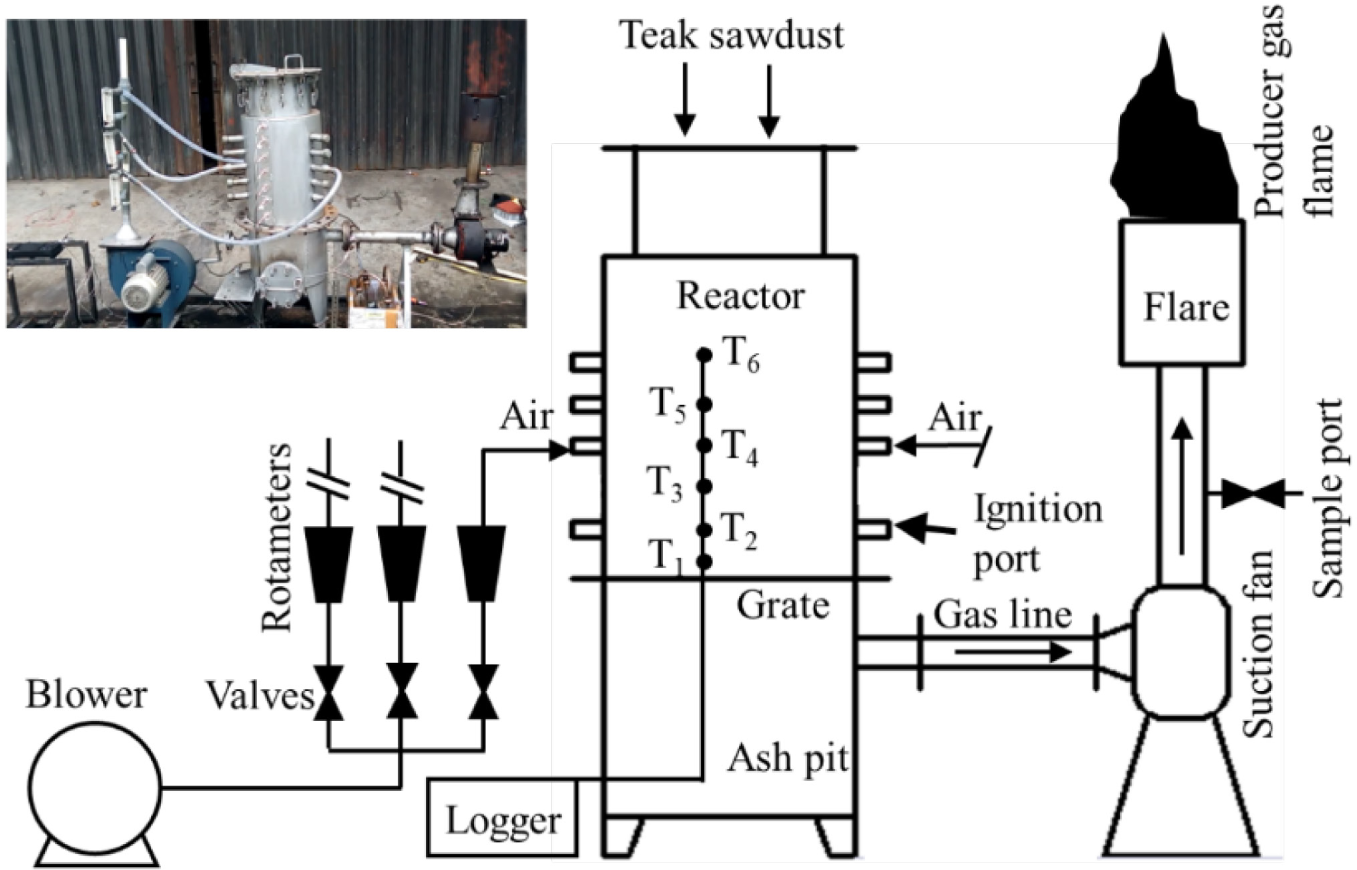
In the present work, the performance of the gasifier fed by teak sawdust is investigated for an equivalence ratio of 0.15, 0.20, 0.25 and tuyere location above grate of 260, 330, and 400 mm. For ø of 0.15, 0.20, and 0.20, 5 kg of teak sawdust requires air flow rates of 3.51, 4.65, and 5.82 m3/h, respectively. Thus, the air flowrates of 1.17, 1.55, and 1.94 m3/h are supplied via each tuyere for an equivalence ratio of 0.15, 0.20, and 0.25, respectively. After 20 minutes of gasification, a producer gas sample is collected from the sampling port near the flare using a vacutainer. The samples are analyzed using Gas Chromatograph SHIMADZU GC-8A to obtain the volume percentages of CO, H2, and CH4 in the combustible gas. Once the gas composition is known, the HHVg and CGE are calculated through the calculation procedure using eq. (5) to eq. (11).
One can evaluate the performance of the gasifier in terms of HHVg and CGE. The HHVg of the producer gas can be calculated using Eq. (5):
(5)
where x1, x3, and x4 are the volume percentages of CO, H2, and CH4. HHV values of CO, H2 and CH4 are 12.71 MJ/Nm3, 12.78 MJ/Nm3, and 39.76 MJ/Nm3, respectively. Meanwhile, the CGE can be obtained using the calculation procedure outlined below [23], also used in Prasad et al.[24].
Mass of producer gas yield per kg of feedstock:
(6)
where:
(7)
and n is the number of moles of the feedstock, while m is the number of moles of the air in the gasification reaction:
(8)
The density of the producer gas:
(9)
where the component densities at NTP are: ρCO = 1.165 kg/Nm3, ρH2 = 0.089 kg/Nm3, ρCH4 = 0.668 kg/Nm3, ρCO2 = 1.842 kg/Nm3, and ρN2 = 1.165 kg/Nm3.
The energy released by producer gas per kg feedstock is:
(10)
Finally, the cold gas efficiency is:
(11)
In this section, an effect of air supply location and equivalence ratio on the thermal performance of the downdraft gasifier fed by sawdust is discussed in terms of the temperature profile, gas volume percentage, HHVg, and CGE.
Figure 3 shows the axial temperature profile in the reactor at the 20th minute gasification time. Uniform temperature distribution occurs at ø of 0.20 for all T-G. For all ø and T-G values, maximum gasification temperature occurs at the height of 120 mm from the grate. This result indicates that the burning front remains where gasification is initiated, 120 mm above the grate. However, several graphs observed maximum temperatures at the height of 50 mm from the grate. The maximum temperature at that location may be due to the oxidation heat in the gas transported directly by the suction fan to the flare.
Axial temperature profiles of the reactor
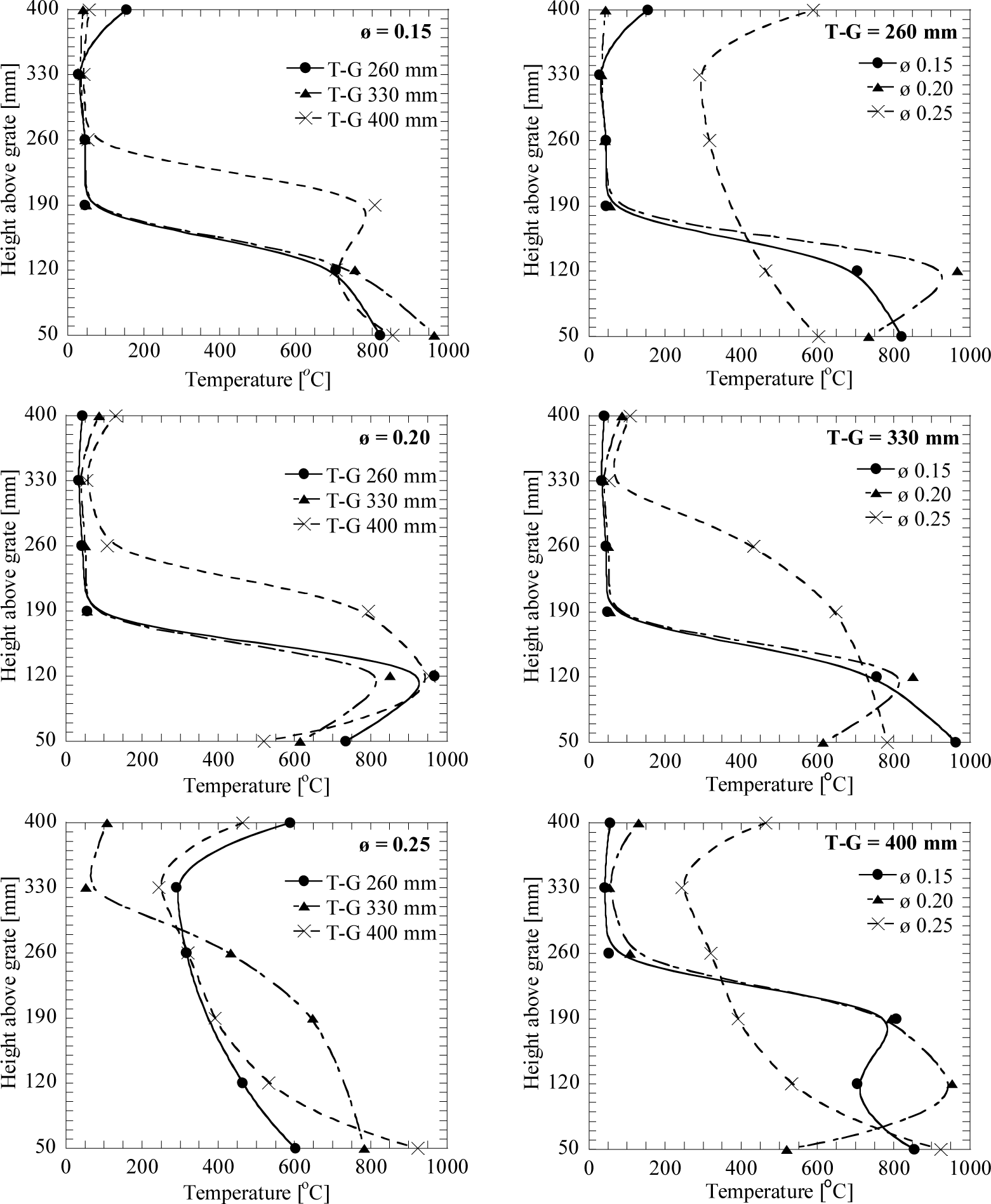
Accumulating heat in the drying zone causes high temperatures in that zone, as can be seen at an equivalence ratio of 0.25. Gasification temperature is related to the equivalence ratio [4]. Increasing the equivalence ratio means more oxygen available during the oxidation process, resulting in better oxidation processes that release more heat for gasification. However, the equivalence ratio cannot be set too high since the gasification process will shift to combustion.
The volume percentage of CO, H2, and CH4 at the 20th minute of gasification is indicated in Figure 4. The figure shows that the volume percentage of CO is the highest for all ø and T-G values. The reason is that the Water-Gas reaction is the fastest among other reduction reactions; thus CO percentage is the highest in the producer gas. On the other hand, methane formation is the slowest reaction; hence much less CH4 is formed during gasification.
Volume percentage of CO, H2, and CH4
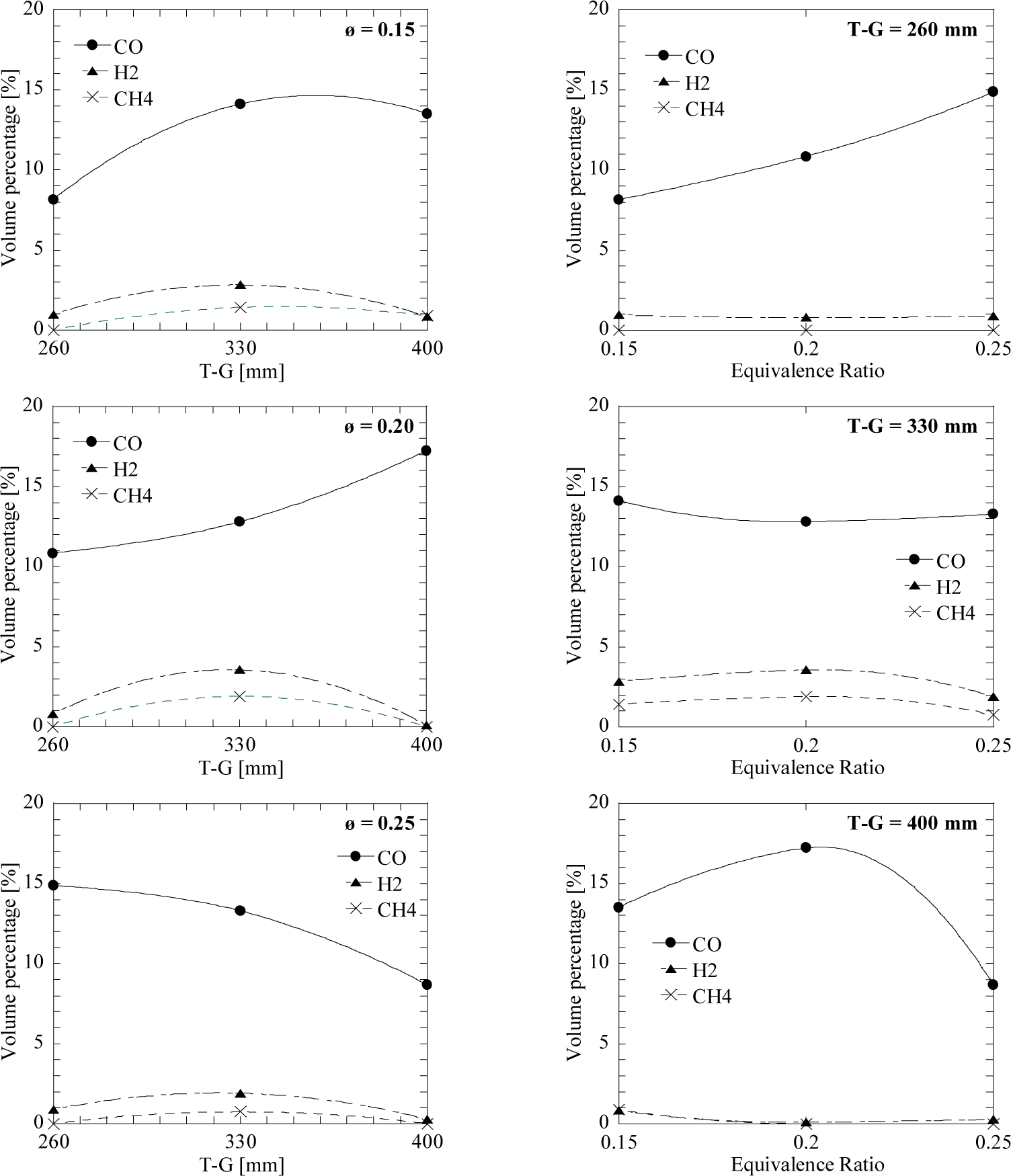
Meanwhile, Figure 5 presents the HHVg and CGE of the gasifier. The highest volume percentage of H2 causes the maximum values of HHVg and CGE to occur at ø of 0.20 and T-G of 330 mm. The maximum HHVg and CGE are 2.84 MJ/Nm3 and 66.36%, respectively. The CGE obtained in the present work is nearly similar to CGE obtained by Wander et al. [24], which is 67.67%.
Higher heating value of the gas and cold gas efficiency
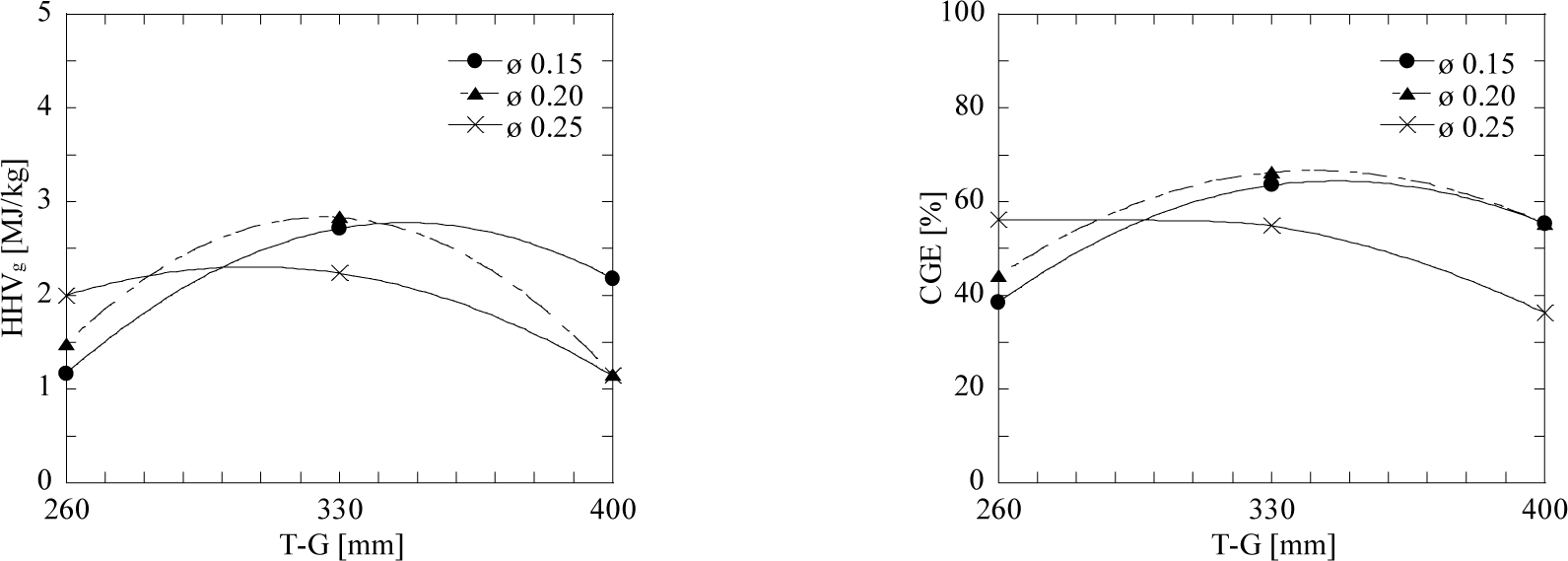
Gasification of teak sawdust waste has been performed successfully. The waste of teak sawdust is suitable as the feedstock of the small-scale downdraft gasifier with proper selection of equivalence ratio and tuyere distance above the grate. The maximum higher heating value of the producer gas is 2.84 MJ/Nm3, and the maximum cold gas efficiency of the gasifier is 66.36%. These results have been obtained at an equivalence ratio of 0.20 and a tuyere distance of 330 mm above the grate.
The authors sincerely thank the Directorate of Research − Universitas Gadjah Mada, which provides financial support for conducting the Post-Doctoral Program 2021-2022 (contract number: 6308/UN1/DITLIT/DIT-LIT/PT/2021) at the Department of Mechanical and Industrial Engineering, Faculty of Engineering, Universitas Gadjah Mada.
CGE |
cold gas efficiency |
[%] |
Eg |
energy released by producer gas per 1 kg feedstock |
[MJ/kg] |
HHVf |
higher heating value of the feedstock |
[MJ/kg] |
HHVg |
higher heating value of producer gas |
[MJ/Nm3] |
MW |
molar mass of a gas component |
[kg/mol] |
m |
number of moles of air |
|
mg |
mass of producer gas |
[kg] |
n |
number of moles of feedstock |
|
T-G |
tuyere-grate distance |
[mm] |
x1 |
volume percentage of carbon monoxide |
[%] |
x2 |
volume percentage of carbon dioxide |
[%] |
x3 |
volume percentage of hydrogen |
[%] |
x4 |
volume percentage of methane |
[%] |
x5 |
volume percentage of nitrogen |
|
ø |
equivalence ratio |
Greek letters |
||
---|---|---|
ρ |
density |
[kg/m3] |
ρg |
density of producer gas |
[kg/m3] |
Abbreviations |
|
---|---|
adb |
air dried basis |
NTP |
Normal Temperature and Pressure |
- , State of the art report for small scale (to 50 kW) gas producer - engine systems, University of California, 1981
Gasification of biomass wastes in an entrained flow gasifier: Effect of the particle size and the residence time ,Fuel Processing Technology , Vol. 91 (6),pp 681–692 , 2010, https://doi.org/https://doi.org/10.1016/j.fuproc.2010.01.018
, - , Biomass Gasification and Pyrolysis, Pratical Design, Elsevier Inc., 2010
Effect of design and operating parameters on the gasification process of biomass in a downdraft fixed bed: An experimental study ,International Journal of Hydrogen Energy , Vol. 39 (11),pp 5625–5633 , 2014, https://doi.org/https://doi.org/10.1016/j.ijhydene.2014.01.130
, Experimental studies of biomass gasification with air ,Journal of Natural Gas Chemistry , Vol. 21 (4),pp 374–380 , 2012, https://doi.org/https://doi.org/10.1016/S1003-9953(11)60379-4
, Gasification of biomass with oxygen-enriched air in a pilot scale two-stage gasifier ,Fuel , Vol. 150 ,pp 386–393 , 2015, https://doi.org/https://doi.org/10.1016/j.fuel.2015.02.056
, System characteristics and performance evaluation of a trailer-scale downdraft gasifier with different feedstock ,Bioresource Technology , Vol. 108 ,pp 264–273 , 2012, https://doi.org/https://doi.org/10.1016/j.biortech.2011.12.105
, Effect of operating and design parameters on the gasification/combustion process of waste biomass in fixed bed downdraft reactors: An experimental study ,Fuel , Vol. 96 ,pp 487–496 , 2012, https://doi.org/https://doi.org/10.1016/j.fuel.2012.01.064
, Experimental studies on producer gas generation from wood waste in a downdraft biomass gasifier ,Bioresource Technology , Vol. 100 (12),pp 3127–3133 , 2009, https://doi.org/https://doi.org/10.1016/j.biortech.2009.01.024
, Influence of particle size on performance of a pilot-scale fixed-bed gasification system ,Bioresource Technology , Vol. 119 ,pp 15–21 , 2012, https://doi.org/https://doi.org/10.1016/j.biortech.2012.05.085
, Gasification and power generation characteristics of woody biomass utilizing a downdraft gasifier ,Biomass and Bioenergy , Vol. 35 (10),pp 4215–4220 , 2011, https://doi.org/https://doi.org/10.1016/j.biombioe.2011.07.008
, Biomass gasification in a downdraft gasifier with a two-stage air supply: Effect of operating conditions on gas quality ,Biomass and Bioenergy , Vol. 61 ,pp 236–244 , 2014, https://doi.org/https://doi.org/10.1016/j.biombioe.2013.12.017
, Performance evaluation of open core gasifier on multi-fuels ,Biomass and Bioenergy , Vol. 30 (6),pp 575–579 , 2006, https://doi.org/https://doi.org/10.1016/j.biombioe.2005.12.006
, Gasifier selection, design and gasification of oil palm fronds with preheated and unheated gasifying air ,Bioresource Technology , Vol. 126 ,pp 224–232 , 2012, https://doi.org/https://doi.org/10.1016/j.biortech.2012.09.018
, Study of the effects of operating factors on the resulting producer gas of oil palm fronds gasification with a single throat downdraft gasifier ,Renewable Energy , Vol. 72 ,pp 271–283 , 2014, https://doi.org/https://doi.org/10.1016/j.renene.2014.07.022
, Experimental study on non-woody biomass gasification in a downdraft gasifier ,International Journal of Hydrogen Energy , Vol. 37 (4935–),pp 4935–4944 , 2012, https://doi.org/https://doi.org/10.1016/j.ijhydene.2011.12.031
, Feasibility study of cashew nut shells as an open core gasifier feedstock ,Renewable Energy , Vol. 31 (4),pp 481–487 , 2006, https://doi.org/https://doi.org/10.1016/j.renene.2005.04.010
, Gasification of hazelnut shells in a downdraft gasifier ,Energy , Vol. 27 (5),pp 415–427 , 2002, https://doi.org/https://doi.org/10.1016/S0360-5442(01)00094-9
, Effect of tuyere distance above grate on propagation front and performance of downdraft gasifier with the feedstock of rice husk ,Renewable Energy , Vol. 134 ,pp 1034–1041 , 2019, https://doi.org/https://doi.org/10.1016/j.renene.2018.11.110
, Gasification of rice husk in downdraft gasifier: The effect of equivalence ratio on the gasification performance, properties, and utilization analysis, of byproducts of char and tar ,BioResources , Vol. 10 (2),pp 2888-2902 , 2015, https://doi.org/https://doi.org/10.15376/biores.10.2.2888-2902
, Gasification and power generation characteristics of rice husk and rice husk pellet using a downdraft fixed-bed gasifier ,Renewable Energy , Vol. 42 ,pp 163-167 , 2012, https://doi.org/https://doi.org/10.1016/j.renene.2011.08.028
, Assessment of a small sawdust gasification unit ,Biomass and Bioenergy , Vol. 27 (5),pp 467–476 , 2004, https://doi.org/https://doi.org/10.1016/j.biombioe.2004.04.003
, Experimental investigation on gasification characteristic of high lignin biomass (Pongamia shells) ,Renewable Energy , Vol. 80 ,pp 415–423 , 2015, https://doi.org/https://doi.org/10.1016/j.renene.2015.02.024
, Pyrolysis and gasification characteristics of Pongamia residue (de-oiled cake) using thermogravimetry and downdraft gasifier ,Applied Thermal Engineering , Vol. 63 (1),pp 379–386 , 2014, https://doi.org/https://doi.org/10.1016/j.applthermaleng.2013.11.005
,